In 1980, Hamish Robertson was a tenured professor at Michigan State. He’d been there since his postdoc in 1971, and he was content. “I want to stress how valued and happy I felt there,” he says. “It was, and still is, an outstanding place.”
But he and his friend and colleague, Tom Bowles, had begun to hatch an idea that would take him far from MSU. They were devising a new experiment to measure the mass of the elusive and perplexingly light neutrino.
Neutrinos are the only fundamental particles whose mass we still don’t know. As their name implies, neutrinos are very, very small. But they outnumber the other fundamental particles by a factor of 10 billion.
Their collective abundance makes it likely that they influenced the formation of structures in the early universe, so knowing their mass is critical to closing gaps in our understanding of the cosmos.
But how do you measure something with a mass so small it approaches zero? Hundreds of physicists, including Robertson, have devoted their careers to solving this problem, and they’re seeing progress. Research projects underway in Europe and the United States fuel a sense of optimism that the task can be accomplished.
To measure directly or indirectly, that is the question
If you were asked to weigh something—your dog for example—how would you do it?
You could set the dog in your car, watch the compression, measure how many inches the car is displaced, then convert that into a measurement of the dog’s mass. You’d need to know the weight of the car, the technical specifications of the shocks, how much air is in the tires, and something about spring constants. That’s an indirect (and hard) way.
Alternatively, you could simply set the dog on a bathroom scale.
The decision on which approach to use, direct versus indirect, depends on what resources you have available. If you don’t have a bathroom scale, an indirect measurement using your car could hypothetically be your best option.
When it comes to neutrinos, scientists have faced a similar situation.
In 1987, astrophysicists interested in the mass of neutrinos got an assist from a rare nearby supernova. The spectral data they collected from the stellar explosion helped them make an indirect measurement that gave them an upper limit on the neutrino mass. As in the car example, they used mathematical models, known quantities, and interactions between many parts of a system to make a calculation.
Cosmologists have also made an indirect measurement by looking for the imprint of neutrino mass on faint radiation in space called the cosmic microwave background, says Diana Parno, an associate professor at Carnegie Mellon University and US spokesperson for the Karlsruhe Tritium Neutrino direct mass experiment, or KATRIN.
“What we would ideally like is an Earth-based measurement of the neutrino mass, and then we can compare that against the cosmological measurement,” Parno says.
So, while cosmologists and astrophysicists look to the sky, experimentalists like Robertson, Bowles and Parno take the direct approach to searching for the neutrino mass. It’s tough—the ghostly particles don’t interact with electromagnetic fields or the nuclear strong force, and they’re so light that gravity barely pulls on them. Experimentalists have to get creative.
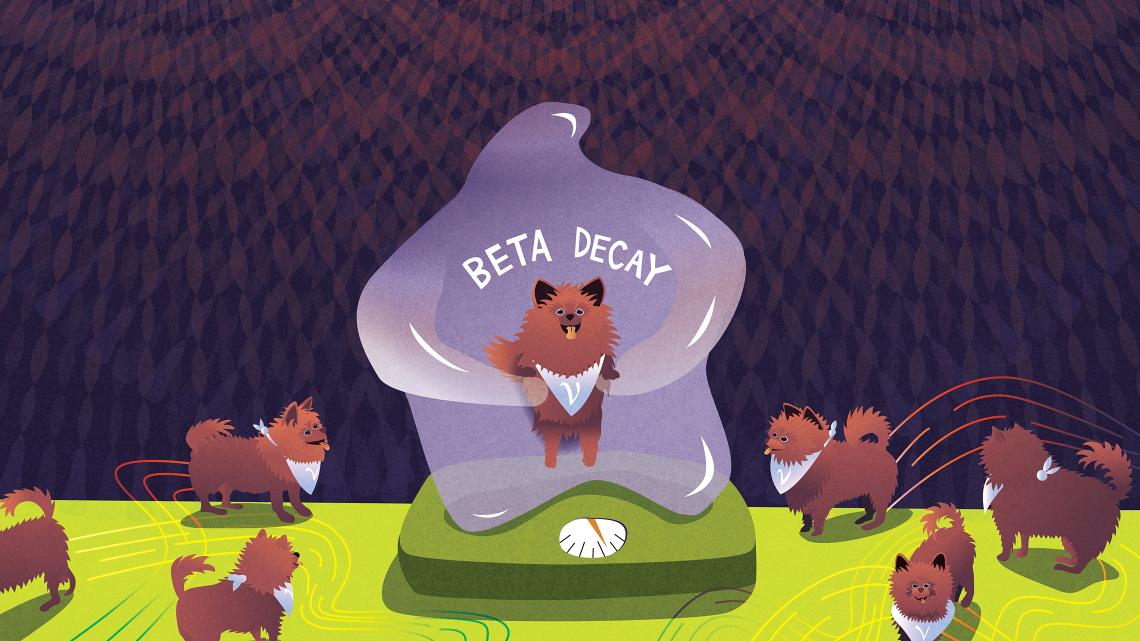
Building a bathroom scale for neutrinos
Back in 1980, Robertson and Bowles developed a new idea for making a direct neutrino mass measurement.
But there were only a few places that had the equipment, the funding and other resources required for the experiment they wanted to propose. The US Department of Energy’s Los Alamos National Laboratory was one of them.
Bowles already worked in Los Alamos, and he wanted Robertson to join him. Robertson was tempted. “Since I first was aware of science as a child, I knew about Los Alamos. I always thought it would be the most extraordinary place to be,” he says.
To convince Robertson to take the dive, Bowles invited him to visit the lab. One night he treated Robertson to dinner at Southwest restaurant Rancho de Chimayo. “On the way back, we stopped the car and turned off the lights,” Robertson says. “We got out so I could marvel at the spectacular carpet of stars in the clear mountain air.”
Robertson was sold. He was ready to embark on the journey to measure neutrino mass.
So how does one go about building a bathroom scale for neutrinos? If you have a squirmy dog that won’t sit on the scale, you can weigh yourself alone, then weigh yourself with your pet. The difference between the two is the weight of the dog. Neutrino researchers use that same idea, taking advantage of processes that produce neutrinos.
In a process called beta decay, a neutron in an unstable nucleus transforms into a proton to restore balance. As the neutron becomes a proton, it emits a negatively charged electron—and a neutrino.
Another process that produces a neutrino is electron capture: A proton in an unstable nucleus captures an electron from the inner shell, converts to a neutron, and ejects a neutrino.
In both cases the events produce a very specific amount of energy—you can look it up in a table. That exact amount of energy is the difference between the mass of the parent atom and the mass of the daughter atom. And that energy is shared between the products: the neutrino and the electron in beta decay, or the neutrino and the excited daughter atom in electron capture.
Experimentalists measure energies, hence they can determine the energy taken by the neutrino. Then they take advantage of that old reliable equation E = mc2 and convert the neutrino’s energy to mass.
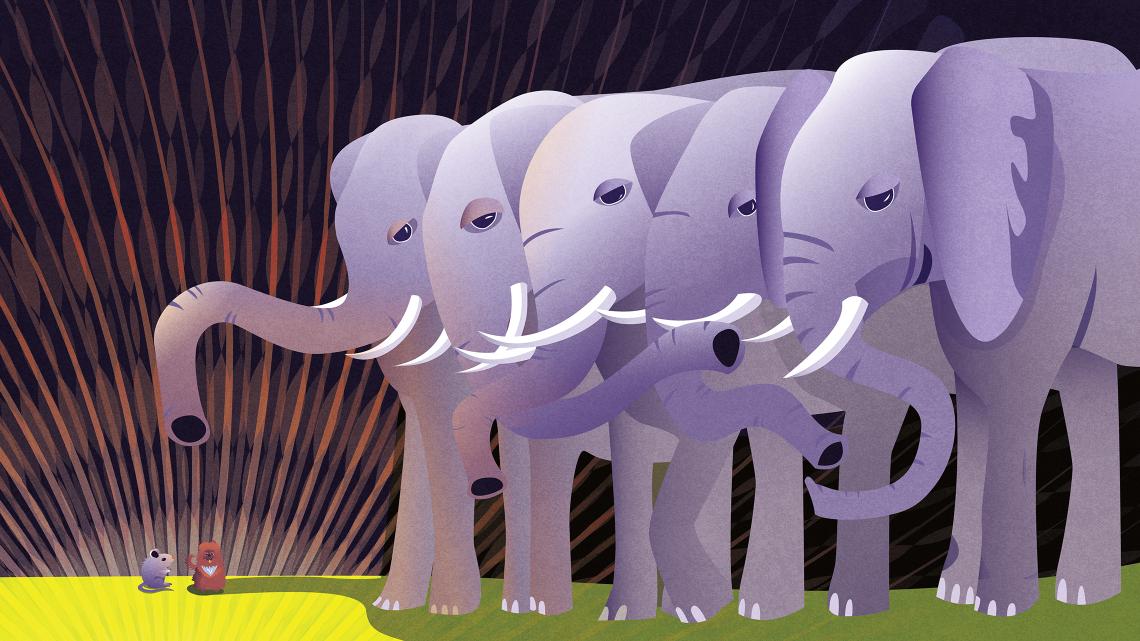
Awkward space in the Standard Model
Why is investigating the mass of neutrinos so alluring to scientists like Robertson and Bowles? Because the unresolved problem has shaken scientists’ understanding of the universe.
The Standard Model of particle physics—our current best explanation of the fundamental forces and particles that make up everything—predicts that neutrinos should have no mass. But oscillation experiments in the 1990s showed that they must have mass.
“Neutrino mass is our first laboratory evidence for physics beyond the Standard Model, and that’s so cool,” Parno says.
As Robertson explains: “Neutrinos are the only matter particles for which the Standard Model made a prediction for what its mass would be. And that prediction was wrong.
“We have examples of things that are not in the Standard Model. Gravity is not in the Standard Model. The mass of quarks is not predicted. But there is only this one case where the Standard Model actually made a prediction, and it got it wrong.”
The upper limit for the neutrino mass, as determined indirectly by cosmology, is roughly one millionth the mass of the next lightest particle, the electron. That’s like the gap between one mouse, which weighs roughly 25 grams, and five elephants, which together weigh roughly 25,000 kilograms. “That’s an enormous gap,” says Parno. “It’s awkward to have this empty space in the Standard Model.”
The gap means that neutrino masses might be special. Or they might not be. But until we have a measurement to work with, we can’t really say anything definitive. So making that measurement is a crucial first step.
Even if researchers achieved a result for the mass of the neutrino, the work would not be done. It would help rule out some theories and models, but there would still be questions, says Patrick Huber, neutrino theorist and director of the Center for Neutrino Physics at Virginia Tech.
“It’s not like once you have the measurement, you immediately select the right model,” he says. “It’s not like every theorist has predicted a certain neutrino mass, and somebody has the right theory once it’s measured. But it would lead to a whole bunch of broader questions.”
Huber has dedicated his career to neutrinos for this exact reason. “If they see the neutrino mass, this will be very exciting. But what would be even more exciting is if they’re not seeing the neutrino mass where they should have seen it, because that means something new is happening,” he says. “Then we are forced to really start thinking anew.”
A ghost worth chasing
Back to the 1980s—Bowles’s persuasion had worked. “The siren song of Los Alamos was too strong to resist,” Robertson says.
Robertson’s wife was also interested in heading out west. As a female nuclear physicist in a male-dominated lab in the ’70s, she had faced an uphill battle to navigate her career. Together, husband and wife secured jobs at the lab and flew down to buy a house. “On the glide path into Albuquerque, I still remember the feeling of elation,” Robertson says. “It was a new beginning.”
A few months later, they officially made the move, with their 6-month-old son in tow.
In 1972 a Swedish physicist named Karl-Erik Bergkvist had declared a new neutrino mass limit: 55 electronvolts. Robertson, Bowles and their colleagues thought they could do better. Their idea was to study beta decay using tritium, a radioactive isotope of hydrogen. Bergkvist had also used tritium, but it was a form implanted into aluminum. Robertson and Bowles wanted to use it in a more pure, gaseous form.
The lab administration was open to their project. “They said, ‘How much money do you need?’ Well, I had no idea,” Robertson says. So he threw out a number. “They said, ‘Okay, fine.’ So that was it. Off we went.”
With that funding—which in the end wasn’t quite enough, but at least got them started—they pushed the limit down to about 10 electronvolts. Importantly, they had proved that tritium decay experiments could work.
In 1988, Robertson shifted gears and joined the Sudbury Neutrino Observatory. In 2001 the group demonstrated neutrino oscillation—a finding that proved neutrinos have mass and that eventually earned the 2015 Nobel Prize in Physics.
Having confirmed that neutrinos have mass, scientists returned to the quest to build a bathroom scale for neutrinos. The KATRIN experiment was forming, and the collaboration members asked everyone who had worked on a tritium experiment in the past to join. Robertson had moved to the University of Washington by then, but he joined the collaboration happily, even offering to design and provide the detector system for the project.
Nestled in Karlsruhe, Germany, the KATRIN experiment now relies on contributions from 150 researchers from seven countries.
Parno is one of those researchers. Like others, she was drawn to the experiment by the thrill of chasing something unknown. “Neutrinos are really weird,” she says. “They keep on surprising and confusing us. I think neutrinos still have a lot to teach us.”
KATRIN is the most advanced direct mass measurement experiment. The KATRIN collaboration published an exciting result in February 2022: Neutrinos must weigh less than 0.8 electronvolts. Oscillation experiments provided a floor, and the KATRIN result provides a ceiling. “We are closing in,” Robertson says.
This range is much tighter than the one from the indirect astrophysical supernova measurement. “The supernova limit is about 5.7 electronvolts, about seven times looser than the current KATRIN limit,” Parno says.
And it’s close to the limit from cosmological indirect measurements, which is somewhere in the range 0.12 to 0.5 electronvolts, depending which parameters are used in the model, Robertson says.
KATRIN’s 0.8 electronvolt number is the new benchmark for all direct mass experiments, but other inventive scientists are on KATRIN’s heels.
New approaches
In 2009, Joe Formaggio, a professor at the Massachusetts Institute of Technology, and Benjamin Monreal, an associate professor at Case Western Reserve University, had another tritium-based idea, which became the foundation of the neutrino mass experiment known as Project 8.
“They proposed just a really beautiful idea,” Robertson says. “It’s one of those ideas where you say: Oh yeah, I wish I thought of that!”
Robertson has worked with the Project 8 team since its inception. The experiment also uses tritium decay, but Project 8 determines the energy of the emitted electron differently. They measure the frequency of the electron’s cyclotron radiation—the microwave radiation that escapes from charged particles in circular orbit in a magnetic field.
“The amount of power radiated is really very small, but you can measure it,” Robertson says.
Various aspects of the experiment are still being developed and tested. If the new approach works as planned, the Project 8 team hopes to measure the neutrino mass with a sensitivity of approximately 0.04 electronvolts.
The third technique currently under investigation as a way to directly measure neutrino mass uses molecules of the holmium isotope 163Ho. It’s challenging as well, says researcher Loredana Gastaldo, Junior Professor at the Kirchhoff Institute for Physics at the University of Heidelberg and spokesperson of the Electron Capture 163Ho, or ECHo, experiment.
“If you want to learn more about neutrinos, you need to be creative, to find an original and clever method that allows you to really learn something about them,” she says. “The neutrinos don’t give anything up as a present, so you need to sweat a lot to gain a little bit more understanding.”
The ECHo experiment relies on electron capture events in 163Ho. Scientists implant 163Ho ions in microcalorimeters, a totally different type of detector than the ones used in KATRIN and Project 8. “The idea is that if energy is deposited into the detector, there is an increase of temperature … and we can measure this extremely small increase in temperature with very precise thermometers,” Gastaldo says.
Each project is different, but through human ingenuity (and persistence), direct mass measurement experiments are making strides.
Let’s get together
Robertson, who is now a professor emeritus at the Center for Experimental Nuclear Physics and Astrophysics at the University of Washington in Seattle, officially retired in 2017. But he’s still working on Project 8.
The collaboration is younger than KATRIN and, so far, has done only proof-of-concept experiments. The next step is to create a full-blown large-scale detector.
“Some days you get up and you learn something that is just not going to work,” Robertson says. “And you say: Oh my goodness, we’re doomed.
“And then you work for another week or two—or a month or a year—and you talk to your friends, and somebody has an idea, and suddenly the sun comes out again, and that problem is solved, and you move on.
“I still work at this 24/7 because I really love this experiment.”
And he’s not alone. Parno, Gastaldo and Huber are just a few of the hundreds of other neutrino experts who have dedicated their careers to finding the mass of the neutrino. And they all rely on each other.
“We are all learning from all the other groups,” Gastaldo says. “And this makes the collaborations really alive and with super interesting discussions in which all of us are gaining a lot of knowledge.”
To maximize neutrino physicists’ ability to learn from each other, Gastaldo organized a conference, called NuMass, in 2016. The inaugural meeting was a gathering of 40 scientists from the US and Europe, all with different expertise. “The discussions were so deep it was unbelievable,” Gastaldo says.
The group repeated the conference in 2018, 2020 and 2022.
“I think it’s fantastically interesting to have so many different fields of knowledge that turn out to be necessary in order to unlock the secrets of this incredibly lightweight, incredibly rarely interacting particle that somehow shaped the universe,” Parno says.
The optimism of neutrino mass researchers is infectious. They continue, with tangible passion, to push the limits of human ingenuity.
And there’s always room for newcomers.
“You have to have young people because they’re the people who actually can get stuff done,” Robertson says. “There’s this huge group of people. I’m just wandering along jumping from the shoulder of one giant to another. That’s part of what makes science fun.”