On July 21, 2017, a group of dignitaries, scientists and engineers gathered in Lead, South Dakota, to hold a unique groundbreaking ceremony—at a research institution in a former gold mine, about one mile underground. The ceremony marked the beginning of construction for the Deep Underground Neutrino Experiment.
DUNE will study neutrinos, fundamental particles of matter that are abundant across the universe but difficult to catch. Over 100 trillion of them flow harmlessly and undetectably through your body each second.
Neutrinos come in three flavors—electron neutrinos, muon neutrinos and tau neutrinos—and they oscillate between those flavors as they travel. This means that a neutrino first produced as an electron neutrino can become a muon or tau neutrino.
“Imagine you bought strawberry ice cream from an ice cream shop, and by the time you brought it home, it turned into chocolate ice cream,” says Shirley Li, a theoretical physicist at the University of California, Irvine who studies neutrinos. “This is what neutrino oscillation is like. It is extremely bizarre.”
An international team of scientists designed the DUNE experiment, which will be the largest neutrino experiment ever built in the United States, to study this strange behavior.
Knowing more about neutrino oscillation could help scientists answer bigger questions, such as whether neutrinos played a role in the imbalance between matter and antimatter that existed after the Big Bang, without which our universe wouldn’t have any of the stars and planets we see today.
Located at the Sanford Underground Research Facility to shield it from the interference of cosmic rays, DUNE will consist of two huge neutrino detectors, each measuring four stories high and weighing 170,000 tons. Both detectors will sit in the path of the world's most intense beam of neutrinos, which will travel 800 miles through the earth from the US Department of Energy’s Fermi National Accelerator Laboratory near Chicago.
Building DUNE will take a little more than a decade. It will involve an extensive upgrade to Fermilab’s particle accelerator, to provide the neutrino beam, and the excavation of 800,000 tons of rock, to carve out space for the detector at SURF. But these are not the only efforts going on in preparation for DUNE.
To be ready to perform the experiment, scientists must also complete a years-long process of developing the theoretical predictions they need to evaluate its data, work known as phenomenology.
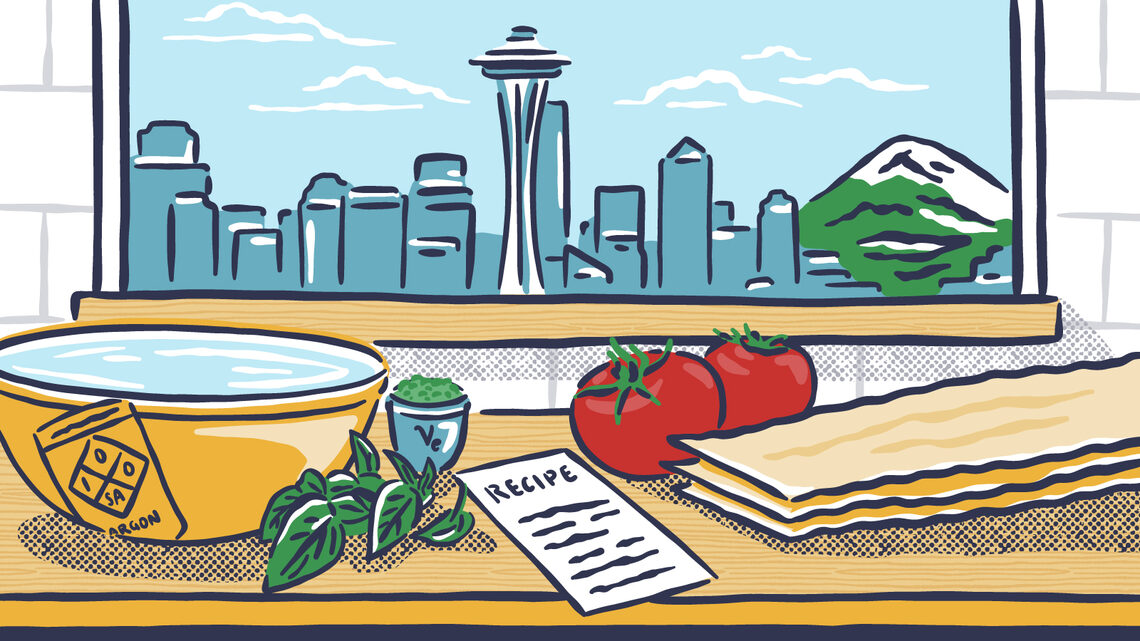
Developing the recipe
Phenomenology is an essential piece of the experimental process. Phenomenology uses fundamental theory to predict what the results of an experiment should look like. If the results stray very far from those predictions, either something is wrong with the experiment, or the experiment has discovered something that was not predicted by the theory.
Let’s say you and four friends want to make lasagna. One friend is more of a fundamental theorist, so she researches every ingredient that can be used to make lasagna and gives you a 500-page report, as any good friend would.
A second friend is more of a model-building theorist. He takes that report and comes up with some possible combinations of ingredients to use, in what order. You, being a phenomenologist, use those models to make precise calculations, determining what amounts of ingredients to use, along with how long to bake the final product.
The fourth friend, being an experimentalist, actually gathers and measures all of your ingredients to your predicted values, sets them up appropriately, and bakes them for the prescribed amount of time.
If the lasagna turns out how it's supposed to, it’s a sign that your theoretical work was correct. If it doesn’t, it could be a sign that there’s something your theory didn’t account for, such as the effect that baking would have on a certain ingredient or how it would change one ingredient to combine it with another.
Making predictions for DUNE
The DUNE detectors will be full of liquid argon. When a neutrino collides with an argon nucleus, the interaction will produce multiple additional particles, which will knock loose electrons from surrounding argon atoms. An electric field will draw these electrons to wire planes or PC boards installed across the detector, which will collect the signals from those drifting electrons. Experimentalists will use the signals to infer the energy, flavor and other properties of the original neutrino. Then they will compare those results to predictions from phenomenologists.
“Understanding the detailed nuclear physics of neutrino collisions with argon is very challenging,” says Michael Wagman, a theorist at Fermilab. “It requires theory that is connected to the real world. As a phenomenologist, I use math and computers to improve models of the collisions we should see in the DUNE experiment.”
Making predictions for DUNE is a particularly difficult task, says Pedro Machado, a theorist at Fermilab. Even though the energy of the collision between a neutrino and a nucleus of liquid argon is generally much lower than the energy of a collision between protons accelerated in the Large Hadron Collider at CERN, phenomenology for DUNE presents different complications than phenomenology for experiments at a particle collider.
“Knowing where collisions happen at the LHC allows for a lot of optimization of the detector,” Machado says. “But because neutrinos interact so little, interactions could happen anywhere in the [DUNE] detectors—and the detectors are huge—making it much harder to optimize them.”
Predictions for DUNE need to deal with both the basic challenges of neutrino detection and the complications of nuclear physics. Multiple theorists are working on making predictions for what should happen in these collisions between neutrinos and nuclei. One significant challenge is combining all of their work into one unified set of values.
An active community of scientists worldwide is working on this by building programs called event generators. Event generators combine predictions from different models of neutrino interactions to make final predictions for what the experiment might see in the detectors.
“There's much work ahead, but we are making a lot of progress,” says Noemi Rocco, a theorist at Fermilab who studies neutrinos. “We need substantial computing resources and collaboration between the nuclear and particle physics communities.”
Phenomenologists for DUNE gather frequently to discuss their advances, most recently at a workshop in Seattle. It is as if multiple chefs have come up with their own lasagna recipes using different lasagna theories. Now, they want to condense all of those recipes into one best lasagna recipe.
Maybe one group has the best sauce, and one has the best pasta. Perhaps one group uses a chicken filling, and another uses mushrooms. “There are lots of variations,” Machado says. “They all have to discuss and come to one conclusion.”