When scientists plan to build a new particle detector, they run simulations to get a picture of what the particle interactions will look like. After constructing and starting up the real thing, they expect a period of tuning, adjusting, fiddling, and fixing to get things running smoothly. They normally don’t expect to turn the detector on and see particle tracks of a quality that exceeds their idealized simulations, especially when it is a prototype detector.
And then there is ProtoDUNE.
“It was fantastic, with neater tracks and less noise from electronics than we expected,” says Flavio Cavanna, a scientist at the US Department of Energy’s Fermilab and the co-coordinator for the first ProtoDUNE detector that came to life this fall. “The entire technology operated as we wanted it to, which is beyond what one can dream.”
The Deep Underground Neutrino Experiment (DUNE) is an international endeavor to unlock the mysteries of particles called neutrinos, which could hold the key to one of the biggest unsolved mysteries in physics: why matter exists in the universe. While the DUNE detector modules ultimately will be 20 times larger, this first prototype detector, ProtoDUNE-SP, is still the largest liquid-argon neutrino detector ever brought to life – and a crucial step in making sure DUNE will work as expected.
For Cavanna and hundreds of others from DUNE institutions in North America, Latin America, Europe, and Asia, these exceptional results are the culmination of three years of hard work and fitful nights. In that short stretch of time, an international team of people had to coalesce; transform a chunk of land into an experimental facility; construct buildings, infrastructure, and an enormous cryogenic container; and design and fabricate the pieces for a house-sized detector that would be assembled inside of that container like a ship in a bottle.
“You build a detector, but never know if it really works until you see the first track,” says Roberto Acciarri, assembly and run coordinator for the detector at CERN, the European Center for Nuclear Research. “You always have that small doubt inside your heart: Is this really going to work?”
A late-night phone call
In December 2015, Cavanna was in Japan for work. One night, he received a call from Fermilab’s director asking if he would like to be a coordinator on a new detector. Only a few things at the time were certain, Cavanna says.
First, the new experiment had been approved by CERN and Fermilab, and CERN agreed that it could live at the Neutrino Platform, a brand-new experimental facility. The detector would use argon, an element found in the air we breathe that becomes a liquid at very cold temperatures. It would serve as one of two test beds (both called ProtoDUNE) for technologies to be used in the Fermilab-hosted Deep Underground Neutrino Experiment.
Second, while many institutions from around the world had formally signed on to participate in DUNE, a full-fledged scientific community dedicated to ProtoDUNE had yet to be identified and organized – and was essential to building a detector of such huge size and scope.
Finally, there was a looming deadline: The detector ideally would be up and running before the start of the long shutdown of the particle accelerator complex at CERN in fall 2018. This was the only way the team could use CERN’s proton beam to make additional measurements in the detector.
“The schedule was tight,” says Fermilab scientist Gina Rameika, the construction coordinator for ProtoDUNE. “Everyone knew the schedule was almost impossible. We had to get it installed and buttoned up in order to get it filled [with liquid argon] and take beam.”
So they got to work.
Build it bigger
Putting together the world’s biggest liquid-argon detector required smart minds and helping hands. The first step was identifying and convincing scientists and engineers willing to make ProtoDUNE-SP the center of their world for the coming three years, working together as a global team.
“DUNE is conceived and set up as an international project. It’s planetary,” Cavanna says. People signed on to ProtoDUNE-SP from institutions in North America, Europe, Latin America, and Asia. “ProtoDUNE was a prototype of DUNE technologically, but also from this collaborative structure perspective.”
Of course, ProtoDUNE-SP is not the first liquid-argon detector ever constructed. The technology was pioneered at the large scale for the ICARUS detector, which ran from 2010 to 2013 at the Italian National Institute of Nuclear Physics’ Gran Sasso National Laboratory under the leadership of Nobel laureate Carlo Rubbia. The team could also look to other liquid-argon experiments, such as MicroBooNE and LArIAT at Fermilab.
“We had a solid foundation from previous efforts, but DUNE will take liquid-argon technology to the yet unexplored multi-kiloton scale,” says CERN’s Francesco Pietropaolo, convener of the ProtoDUNE high-voltage consortium. “We knew ProtoDUNE would be essential for us to test new technologies and see how we could scale up to the much larger volume needed for DUNE.”
As groups around the globe made design decisions and mock-ups and eventually started fabricating their individual pieces, construction got under way at CERN, with Marzio Nessi as the head of CERN’s Neutrino Platform. The wooded plot of land was transformed as crews extended a nearby facility and carved out a giant pit where ProtoDUNE-SP and its sister detector would live. CERN’s experts built a beamline that would funnel in the particle beam.
Within the pit, welders got to work on a gigantic red steel frame, the external structure that would house the container with the detector components and the liquid argon needed to capture particle interactions. Researchers adapted algorithms and built up the software and hardware that would capture the electronic signals when a particle from the beam smashed into an argon nucleus in the detector. Things started coming together.
Pieces of ProtoDUNE-SP began flowing in from around the world. Researchers from the Physical Sciences Lab at the University of Wisconsin-Madison sent the first of six crucial components called anode plane assemblies, special panels of wire that record particle interactions. On July 14, 2017, Cavanna sat in front of that very large APA shipping box at CERN and wondered how they would produce, test, and install five more before their stringent deadline just a bit more than a year ahead. The teams went into high gear. Detector parts came faster and faster.
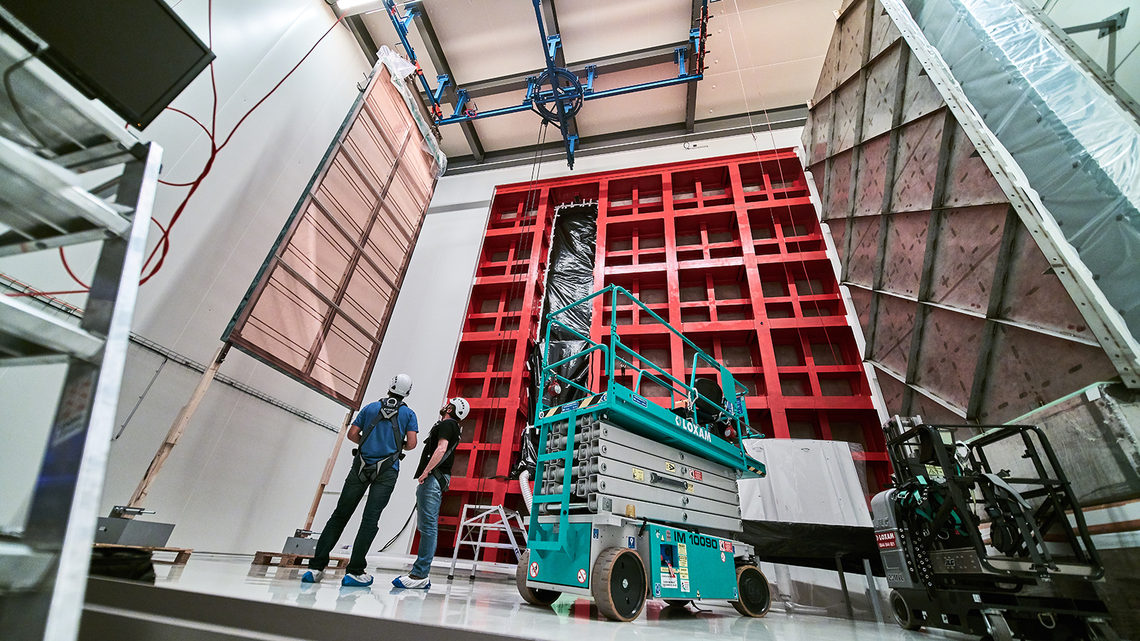
An anode plane assembly (APA) is prepared for installation into ProtoDUNE.
“There were rumors that we would never make the schedule and we’d be lucky to put in two APAs,” Rameika says. “We were driven to prove we could deliver all of them, and we did.”
Shipments of APAs from Wisconsin and a group of universities supported by the UK Science and Technology Facilities Council arrived. Teams tested detector components, then slid them through a narrow opening in the steel structure to an inner space where only a few people could work at a time. Fragile photo-sensors were added into the APAs. Electronics came together, cables were strung, and soon the temporary entrance in the side of the container was welded shut.
To complete the final installation, technicians slid into the detector through a one-meter diameter manhole in the roof. CERN’s cryogenic experts filled the detector with 800 metric tons of liquid argon and turned on the purifiers, letting the detector cycle the clear liquid and remove any stray bits of non-argon material. The components within were cut off from any rescue should something go wrong. When the filling was completed almost eight weeks later, ProtoDUNE scientists checked the equipment. CERN’s particle accelerator operators sent streams of protons toward the detector, and researchers turned up the power on the high-voltage system.
“The run started with this critical step that was keeping me up every night for three years,” Cavanna says. “It was the moment of bringing the detector to life, and I didn’t know what to expect.”
Current flowed through the high-voltage system at a whopping 180,000 volts – exactly what it was supposed to do, “like it would be written in a textbook,” Cavanna says. Particle tracks showed up on the display, and soon after, celebratory champagne flowed in the ProtoDUNE control room at CERN. People around the world toasted their victory over video connections.
Non-stop data
When you have limited beam time, every second counts. Particles from the accelerator bombarded the ProtoDUNE detector 24 hours a day, seven days a week, but the deadline for shutting down the beam to prepare for a 2-year-long upgrade to the CERN accelerator complex loomed large.
“You basically set aside your life when there’s beam,” Acciarri says. “You are always thinking and making sure everything is working properly. It’s very stressful.”
After beam and detector tuning, between October 2 and November 12, ProtoDUNE-SP researchers collected more than 4 million gorgeous images of particle interactions. Members from participating institutions took shifts in the control rooms to make sure systems were operating as they should and watched the data roll in.
“This is the first time we have had a live, 3-D event display for a liquid-argon detector,” says Tingjun Yang, co-convener for the data reconstruction and analysis group. Starting with the software used for the data analysis of another neutrino experiment, MicroBooNE, multiple groups collaborated to create a package to convert live data into the right format for quick, 3-D images that researchers on shift could use to monitor the detector.
“We recognized this was a really powerful tool that DUNE will want to use,” Yang says. “We developed it, and the data worked. It was very beautiful.”
This 3-D display shows a particle event at ProtoDUNE. The video shows the full size of the ProtoDUNE-SP detector (white box) and the direction of the particle beam (yellow arrow). Particles from other sources (such as cosmic rays) can be seen throughout the white box, while the red box highlights the region of interest: in this case, an interaction resulting from the particle beam passing through the detector. Event information, such as the momentum of particles in the beam and time of interaction, are located in the lower left corner. For curious minds that want to play with the interface, a selection of 3-D events from ProtoDUNE-SP are available in an online gallery developed by Chao Zhang of Brookhaven National Laboratory.
Over the course of the run, researchers collected data about all sorts of different particles that might come out of a neutrino interaction in a detector: pions, kaons, photons, electrons, protons, and more. Because ProtoDUNE-SP sits on Earth’s surface, it also sees a high number of cosmic rays that the final DUNE detector won’t see from its mile-deep home at the Sanford Underground Research Facility in South Dakota.
“It makes ProtoDUNE a great stress test for the detector and reconstruction capability,” Yang says. If the software tested at ProtoDUNE can handle the high number of particle interactions, it will be almost overqualified for the more serene environment of DUNE. Fermilab’s accelerator complex will send trillions of neutrinos through 800 miles of earth, but the far detectors will see only a handful every day. However, ProtoDUNE-SP’s robust data handling capabilities are needed to search for rare subatomic phenomena, such as the hypothesized decay of protons. It also ensures that DUNE can handle thousands of neutrino interactions in a few seconds if, say, a star explodes in the Milky Way.
ProtoDUNE-SP also collected particles at the full range of energies DUNE expects to see: from 1 to 7 gigaelectronvolts (GeV). In fact, data-taking went so smoothly at these planned energies that researchers even had extra time to capture lower-energy particles, from 0.3 GeV up to 1 GeV. With precise control over the beam, scientists were able to carefully study how particles interact with the argon atoms – important physics studies in their own right – and test the detector components within.
“The technology is here, and it’s ready for DUNE,” Acciarri says. “We’ll take this opportunity to change a few things, both on the hardware and software side, to make things go even smoother, but I do believe we reached more than what we were expecting or asking for this detector to show.”
Looking ahead
There is plenty still to do, Acciarri notes. DUNE will run for decades, so researchers aim to operate the prototype for as long as possible to monitor how the pieces of the detector fare over time. There also are plans for a series of tests on all the subsystems: things like the light detection system, electronics, and high-voltage system. They plan to test their models of fluid dynamics, seeing how the argon circulates in the detector, and how each subsystem affects the others. Two consortia are already working on improvements for the crucial anode plane assemblies for DUNE. On the software side, researchers will work to improve the stability of the system and the speed at which it captures events. And scientists are working to complete a second ProtoDUNE detector at CERN, known as the dual-phase ProtoDUNE.
DUNE already has around 1,000 members from more than 30 countries and continues to grow. With all the ongoing planning, construction, and testing taking place around the world, the team of DUNE scientists and engineers, it seems, will have a busy and collaborative 2019.
“That was something that was quite important beyond the technological success," Cavanna says. “Technology without the right people is just a piece of material that is dead. We grew a community that will bring DUNE to life.”

An APA hangs from a crane in CERN’s Neutrino Platform
Editor's note: A version of this article was originally published by Fermilab.