Studies of the cosmos indicate that most of the matter that makes up the universe is dark matter—so called because it does not emit or reflect light. But even ordinary matter, the matter that makes up everything we can see, can be difficult to find.
“If you look with an optical telescope, you're seeing only a tiny fraction of the total matter,” says Emmanuel Schaan, a physicist at the Department of Energy's SLAC National Accelerator Laboratory.
Some matter, like dust and gas at the very edges of a galaxy or galaxy cluster, doesn't emit any light of its own, and very little light shines on it. By contrast, other matter is too close to light sources to be seen. The light from matter that sits close to the heart of galaxies and galaxy clusters is hidden behind the brighter light of other objects like stars.
The result is that only a small percentage of what’s considered visible matter is actually visible. “Ordinary matter is only 5% of the total energy in the universe,” Schaan says, “and if you look at stars within galaxies, you're only seeing 5 to 10% of the ordinary matter.”
Detecting that hidden ordinary matter, also called baryonic matter, will become increasingly important in years to come, Schaan says. “If we don't know where the baryons are, then we cannot properly analyze precision data from experiments like LSST,” the Rubin Observatory's Legacy Survey of Space and Time. Data from these experiments “contain a lot of precious information that will help us learn about dark energy, dark matter, inflation and neutrinos, but if we can't properly model it, we'll have to throw that data away.”
Fortunately, astrophysicists are used to searching for things they can’t see. Just as they use a variety of methods to learn about the distribution of dark matter in the universe, they have identified ways to learn about the distribution of ordinary matter as well.
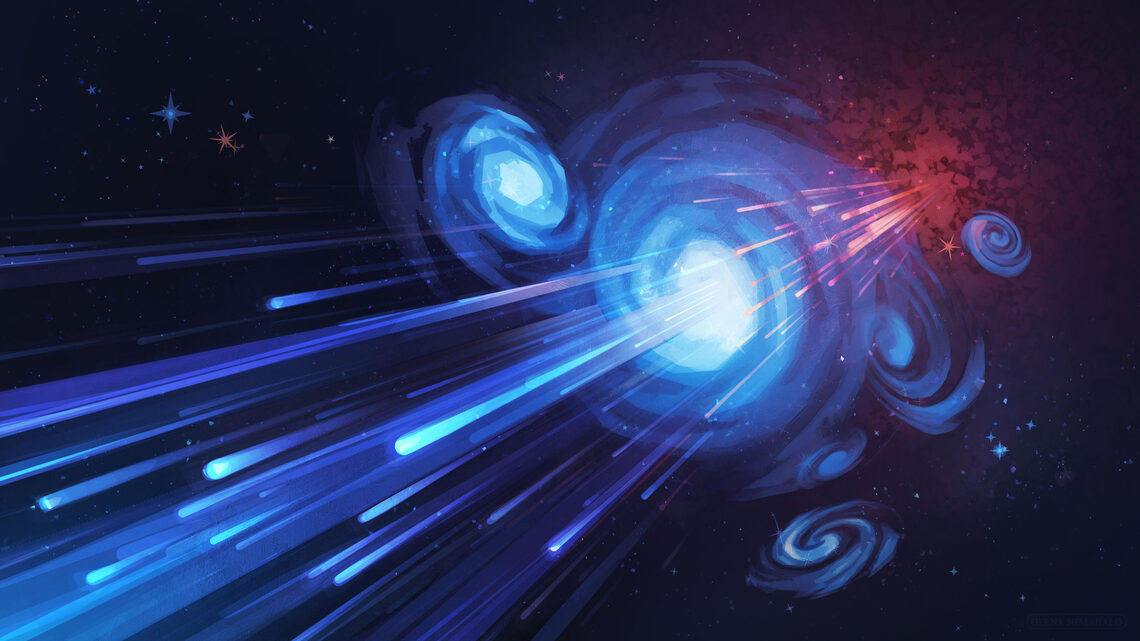
Missing matter and the cosmic backlight
Cosmologists have a couple of ways to calculate how much ordinary matter there is in the universe. One way is to look at the relative amounts of hydrogen, deuterium, helium and other light elements and isotopes that exist today. According to the theory of Big Bang nucleosynthesis, these ratios depend on the number of baryons in the universe, so physicists can use them to infer how much ordinary matter there is.
An independent estimate comes from the cosmic microwave background, the oldest light in the universe. That light is left over from a time when the universe cooled from an extremely hot plasma soup of electrons, protons and neutrons down to a more ordinary mix of hydrogen and helium. Tiny variations in the CMB reveal how that matter moved around and clumped up in the very early universe. From those observations, researchers can infer the mix of matter and dark matter.
Either way, physicists have concluded that around 16% of the matter in the universe is ordinary, while the rest is dark matter.
Yet when physicists catalogued all the ordinary matter they could find, in dust and gas and stars in galaxies, they could account only for a little more than half what the other measurements suggested should be there, Schaan says.
Scientists have described this as the missing baryon problem.
Over the last decade, researchers discovered that much of the missing matter started out in galactic centers and got blasted out to the galaxies' extreme edges by such violent phenomena as supernovae and active galactic nuclei, leading some to declare the missing baryon problem solved.
Still, researchers like Schaan want to know more about exactly how that matter is distributed in and around galaxies. In part, that's to better understand galactic astrophysics for its own sake, but it's also because understanding where the matter is and how it got there will inform the analysis of data from experiments like LSST, along with others that are trying to map out dark matter and dark energy.
To map out the rest of the ordinary matter, then, Schaan and colleagues including Stefania Amodeo, Simone Ferraro and Nicholas Battaglia, along with other teams, have taken to looking for shadows. Using the CMB as a backlight, they look for a kind of galactic watermark whereby the heat and motion of dust and gas at the edges of galaxies boost the energy of the CMB light. These effects, known collectively as the Sunyaev-Zeldovich effects, can reveal both where the dust and gas are and also their speed and energy—thus providing essential new details on how it got there.
Schaan says certain flavors of the SZ effect have already been measured fairly well—in particular a variety that reveals how fast dust and gas are moving—but others remain elusive. The hope is that with experiments such as the Atacama Cosmology Telescope gathering more CMB data, researchers will finally be able to get a good picture of the matter surrounding galaxies. “I think this will bloom in the next few years,” Schaan says.
X-raying the universe
While the cosmic-backlight approach should work well for mapping matter at the edges of galaxies, it does have a significant shortcoming: Astrophysicists still want to map the distribution of matter nearer to the heart of galaxies and galaxy clusters, but in those spots, light from stars and other sources will wash out the CMB.
An alternative, says SLAC physicist Steven Allen, is to look instead for X-rays emitted by baryonic matter. Although galactic processes may blow some of that matter away, a considerable amount remains—and it gets very hot. In large galaxy clusters, Allen explains, gas temperatures can reach into tens of millions of degrees, at which point the gas emits a lot of X-rays.
Mapping the distribution of matter in these hot zones gives scientists a way to test their assumptions about how galaxies and galaxy clusters form and evolve.
For the most part, Allen says, the temperature and distribution of matter detected via X-rays is consistent with the idea that matter collapses and heats under the force of gravity. But Allen's team and other X-ray astronomers have discovered that more heating has occurred than physicists would expect from gravitational collapse alone, and active galactic nuclei appear to have provided that heating.
Allen's group has also looked into another use of X-ray data, drawing on X-ray spectroscopy. That method reveals a chemical composition that again points to the material having been blasted around more powerfully and earlier in the history of the universe than scientists had imagined. “[Active galactic nuclei] must have put a lot of energy into the gas, and it must have happened relatively early,” Allen says.
If researchers don't fully understand galaxy formation and evolution, then the assumptions cosmologists make about galaxies may be incorrect and could throw off models of how matter shifted around the universe as it evolved. That could in turn have implications for how cosmologists understand the nature of dark matter and dark energy, which could feed back into astrophysicists' studies of galaxy formation and evolution. “You can't do one without the other,” Allen says.
But investigations like Allen's can help. By looking at X-rays from galaxies and galaxy clusters at different distances, researchers can map out how these objects evolved over time. Since distance is a proxy for how long ago the light we see was emitted, that could help astrophysicists build better models of galaxies that in turn help cosmologists improve their models of the universe.
Making sense of the data
As researchers gather more and more detailed data on what's out there in the universe, theoretical models must keep up, says Chihway Chang, an astrophysicist and cosmologist at the University of Chicago and a former student at SLAC and Stanford University. That’s because this new information is only as valuable as scientists’ ability to translate it.
Chang points to the Dark Energy Survey, a project aimed at mapping out how quickly the universe has expanded over time, as an example. “As we've started to analyze more and more data from DES, we're getting beautiful data with super high signal-to-noise ratio, but you can't use all of it because our theoretical understanding on the smallest scales is very uncertain.”
For that reason, scientists are working to build better models of astrophysical processes considered small-scale (compared to the size of the universe, that is), such as the process of active galactic nuclei spitting matter out to galactic edges, Chang says.
Doing so could help researchers better understand some current tensions in cosmology—for instance, measurements based on the CMB suggest that matter is slightly more prone to clustering than one would expect using measurements based on studies of weak gravitational lensing, or the way the gravitational pull of matter bends light. Or it could reveal entirely new physics that researchers haven't thought of yet.
Despite the challenges, it's an exciting time for the field, says Agnès Ferté, a cosmologist at SLAC. “A few years ago, the most precise information about cosmology came from the cosmic microwave background.”
As researchers have added data on galaxy clustering and different varieties of gravitational lensing, they've learned more and more about the structure and history of the universe.
Adding better models of smaller-scale astrophysical phenomena should help continue the advances, Ferté says, especially as even more detailed surveys from the Rubin Observatory and the European Space Agency's Euclid satellite come on line. Perhaps, she says, researchers will even be able to make more precise tests of the theory of gravity itself or finally discover the nature of dark matter and dark energy.
“I'm extremely excited,” Ferté says. “I think we will discover something new for sure.”