As a massive star dies, expelling most of its guts across the universe in a supernova explosion, its iron heart, the star’s core, collapses to create the densest form of observable matter in the universe: a neutron star.
A neutron star is basically a giant nucleus, says Mark Alford, a professor at Washington University.
“Imagine a little lead pellet with cotton candy around it,” Alford says. “That’s an atom. All the of mass is in the little lead pellet in the middle, and there’s this big puffy cloud of electrons around it like cotton candy.”
In neutron stars, the atoms have all collapsed. The electron clouds have all been sucked in, and the whole thing becomes a single entity with electrons running around side-by-side with protons and neutrons in a gas or fluid.
Neutron stars are pretty small, as far as stellar objects go. Although scientists are still working on pinning down their exact diameter, they estimate that they’re somewhere around 12 to 17 miles across, just about the length of Manhattan. Despite that, they have about 1.5 times the mass of our sun.
If a neutron star were any denser, it would collapse into a black hole and disappear, Alford says. “It’s the next to last stop on the line.”
These extreme objects offer intriguing test cases that could help physicists understand the fundamental forces, general relativity and the early universe. Here are some fascinating facts to get you acquainted:
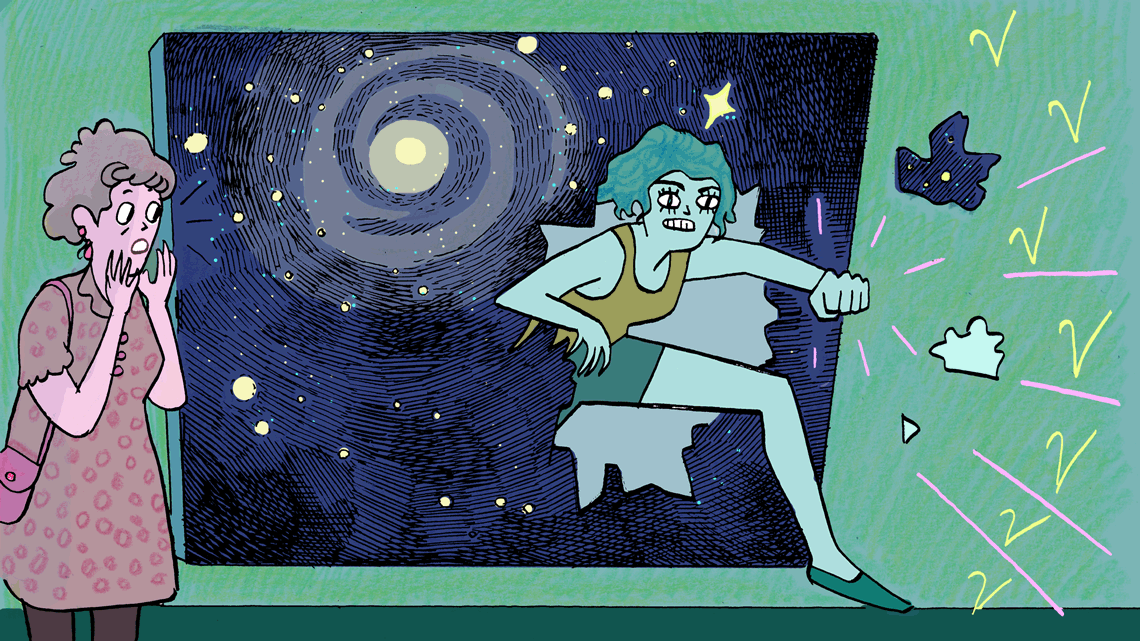
1. In just the first few seconds after a star begins its transformation into a neutron star, the energy leaving in neutrinos is equal to the total amount of light emitted by all of the stars in the observable universe.
Ordinary matter contains roughly equal numbers of protons and neutrons. But most of the protons in a neutron star convert into neutrons—neutron stars are made up of about 95 percent neutrons. When protons convert to neutrons, they release ubiquitous particles called neutrinos.
Neutron stars are made in supernova explosions which are giant neutrino factories. A supernova radiates 10 times more neutrinos than there are particles, protons, neutrons and electrons in the sun.
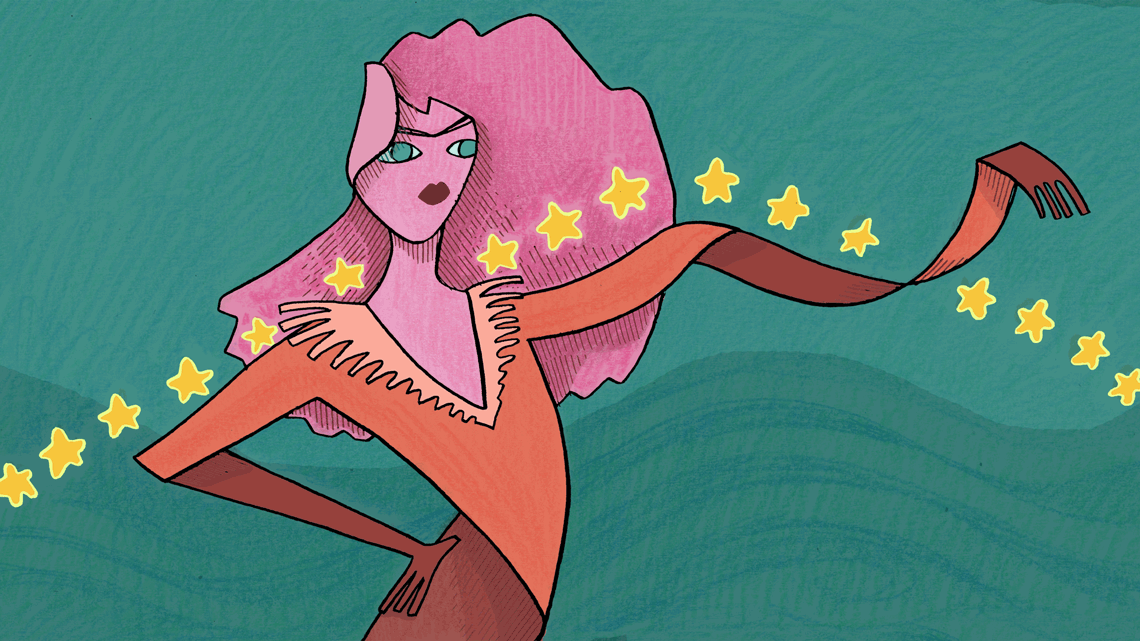
2. It’s been speculated that if there were life on neutron stars, it would be two-dimensional.
Neutron stars have some of the strongest gravitational and magnetic fields in the universe. The gravity is strong enough to flatten almost anything on the surface. The magnetic fields of neutron stars can be a billion times to a million billion times the magnetic field on the surface of Earth.
“Everything about neutron stars is extreme,” says James Lattimer, a professor at Stony Brook University. “It goes to the point of almost being ridiculous.”
Because they’re so dense, neutron stars provide the perfect testbed for the strong force, allowing scientists to probe the way quarks and gluons interact under these conditions. Many theories predict that the core of a neutron star compresses neutrons and protons, liberating the quarks of which they are constructed. Scientists have created a hotter version of this freed “quark matter” in the Relativistic Heavy Ion Collider and the Large Hadron Collider.
The intense gravity of neutron stars requires scientists to use the general theory of relativity to describe the physical properties of neutron stars. In fact, measurements of neutron stars give us some of the most precise tests of general relativity that we currently have.
Despite their incredible densities and extreme gravity, neutron stars still manage to maintain a surprising amount of internal structure, housing crusts, oceans and atmospheres. “They’re a weird mixture of something the mass of a star with some of the other properties of a planet,” says Chuck Horowitz, a professor at Indiana University.
But while here on Earth we’re used to having an atmosphere that extends hundreds of miles into the sky, because a neutron star’s gravity is so extreme, its atmosphere may stretch up less than a foot.
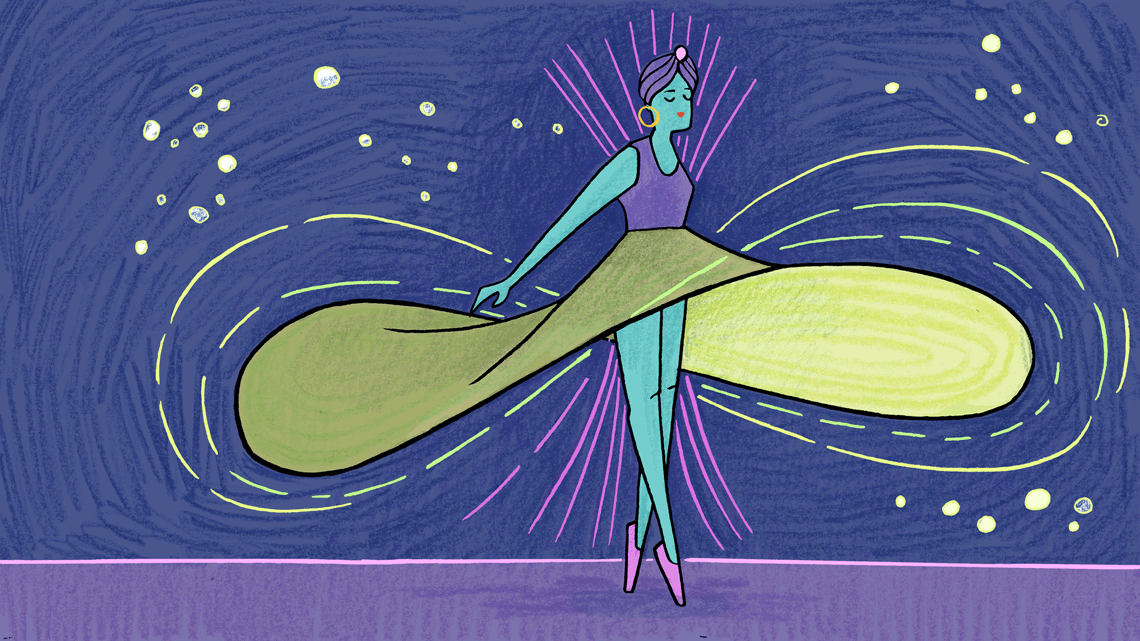
3. The fastest known spinning neutron star rotates about 700 times each second.
Scientists believe that most neutron stars either currently are or at one point have been pulsars, stars that spit out beams of radio waves as they rapidly spin. If a pulsar is pointed toward our planet, we see these beams sweep across Earth like light from a lighthouse.
Scientists first observed neutron stars in 1967, when a graduate student named Jocelyn Bell noticed repeated radio pulses arriving from a pulsar outside our solar system. (The 1974 Nobel Prize in Physics went to her thesis advisor, Anthony Hewish, for the discovery.)
Pulsars can spin anywhere from tens to hundreds of times per second. If you were standing on the equator of the fastest known pulsar, the rotational velocity would be about 1/10 the speed of light.
The 1993 Nobel Prize in Physics went to scientists who measured the rate at which a pair of neutron stars orbiting each other were spiraling together due to the emission of gravitational radiation, a phenomenon predicted by Albert Einstein's general theory of relativity.
Scientists from the Laser Interferometer Gravitational-Wave Observatory, or LIGO, announced in 2016 that they had directly detected gravitational waves for the first time. In the future, it might be possible to use pulsars as giant, scaled-up versions of the LIGO experiment, trying to detect the small changes in the distance between the pulsars and Earth as a gravitational wave passes by.
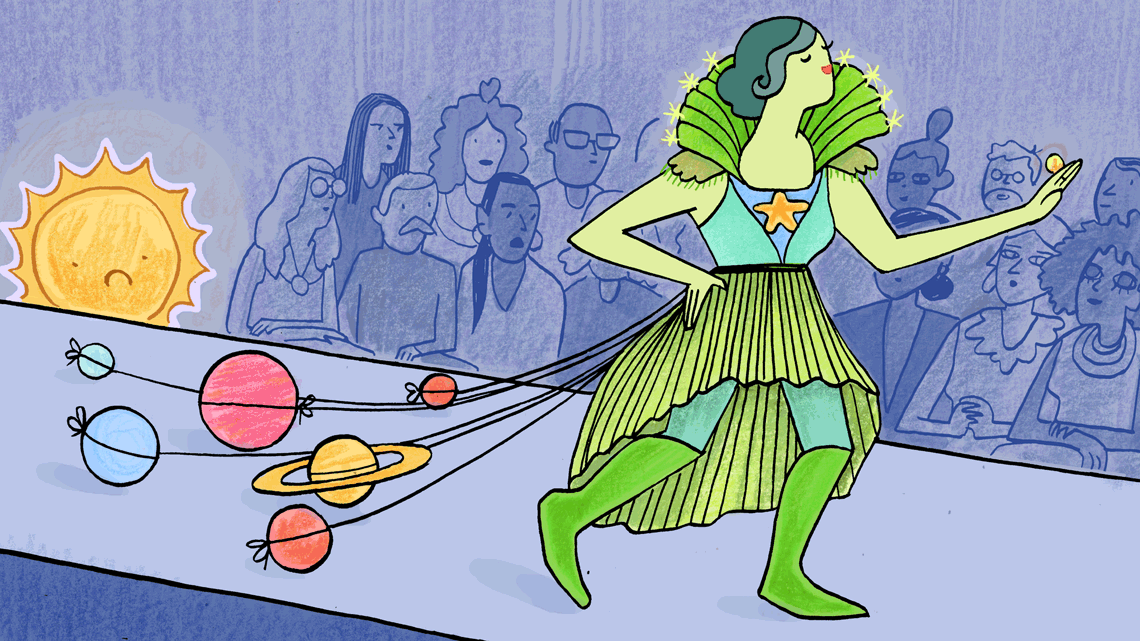
4. The wrong kind of neutron star could wreak havoc on Earth.
Neutron stars can be dangerous because of their strong fields. If a neutron star entered our solar system, it could cause chaos, throwing off the orbits of the planets and, if it got close enough, even raising tides that would rip the planet apart.
But the closest known neutron star is about 500 light-years away. And considering Proxima Centauri, the closest star to Earth at a little over 4 light-years away, has no bearing on our planet, it’s unlikely we’ll feel these catastrophic effects anytime soon.
Probably even more dangerous would be radiation from a neutron star’s magnetic field. Magnetars are neutron stars with magnetic fields a thousand times stronger than the extremely strong fields of “normal” pulsars. Sudden rearrangements of these fields can produce flares somewhat like solar flares but much more powerful.
On December 27, 2004, scientists observed a giant gamma-ray flare from Magnetar SGR 1806-20, estimated to be about 50,000 light years away. In 0.2 seconds the flare radiated as much energy as the sun produces in 300,000 years. The flare saturated many spacecraft detectors and produced detectable disturbances in the Earth’s ionosphere.
Fortunately, we are not aware of any nearby magnetars powerful enough to cause any damage.
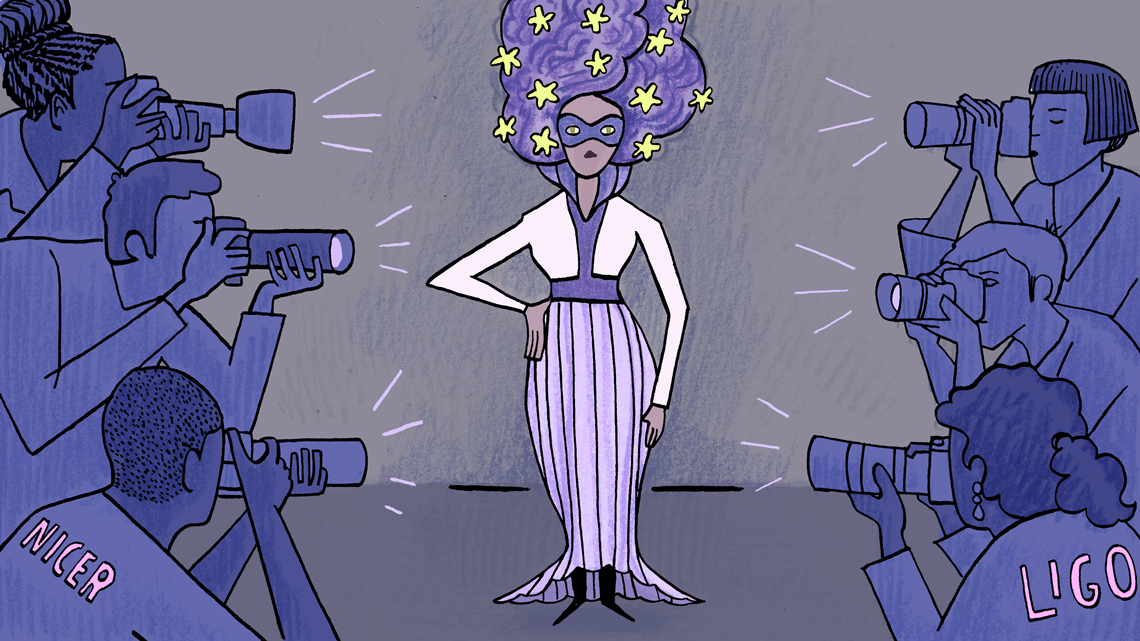
5. Despite the extremes of neutron stars, researchers still have ways to study them.
There are many things we don’t know about neutron stars—including just how many of them are out there, Horowitz says. “We know of about 2000 neutron stars in our own galaxy, but we expect there to be billions more. So most neutron stars, even in our own galaxy, are completely unknown.”
Many radio, X-ray and optical light telescopes are used to investigate the properties of neutron stars. NASA’s upcoming Neutron Star Interior Composition ExploreR Mission (NICER), which is scheduled to attach to the side of the International Space Station in 2017, is one mission devoted to learning more about these extreme objects. NICER will look at X-rays coming from rotating neutron stars to try to more accurately pin down their mass and radii.
We could also study neutron stars by detecting gravitational waves. LIGO scientists hope to detect gravitational waves produced by the merger of two neutron stars. Studying those gravitational waves might clue scientists in to the properties of the extremely dense matter that neutron stars are made of.
Studying neutron stars might help us figure out the origin of the heavy chemical elements, including gold and platinum, in our universe. There’s a possibility that when neutron stars collide, not everything gets swallowed up into a more massive neutron star or black hole, but instead some fraction gets flung out and forms these heavy nuclei.
“If you want to use the lab of 24th or 25th century,” says Roger Romani, a professor at Stanford University, “then studying neutron stars is a way of looking at conditions that we cannot produce in labs on Earth.”