Underneath the vast, frozen landscape of the South Pole lies IceCube, a gigantic observatory dedicated to finding ghostly subatomic particles called neutrinos. Neutrinos stream through the Earth from all directions, but they are lightweight, abundant and hardly interact with their surroundings.
The IceCube detector consists of an array of 86 strings festooned with more than 5000 sensors, like round, basketball-sized Christmas lights. They reach more than 2 kilometers (more than 1 mile) down through layers of Antarctic ice that have accumulated over hundreds of thousands of years.
A small fraction of the neutrinos that pass through the ice collide with its atoms and spit out showers of particles, some of which can be spotted by IceCube’s sensors as sparks of blue light. By probing the light patterns, scientists can identify and assess the elusive particles, some of which originate beyond our solar system.
In July 2019 the IceCube collaboration announced that the US National Science Foundation had granted it $23 million to put toward a $37 million upgrade, with additional financial support coming from Michigan State University, the University of Wisconsin–Madison, and agencies in Germany and Japan.
The upgrade will add seven more strings of sensors to the detector, along with new instruments meant to characterize the ice. This extension will allow physicists to better understand how neutrinos oscillate between their three flavors: electron, muon and tau. Scientists also plan to make more precise measurements of IceCube’s icy interior to get a closer look at neutrinos from far out in the universe.
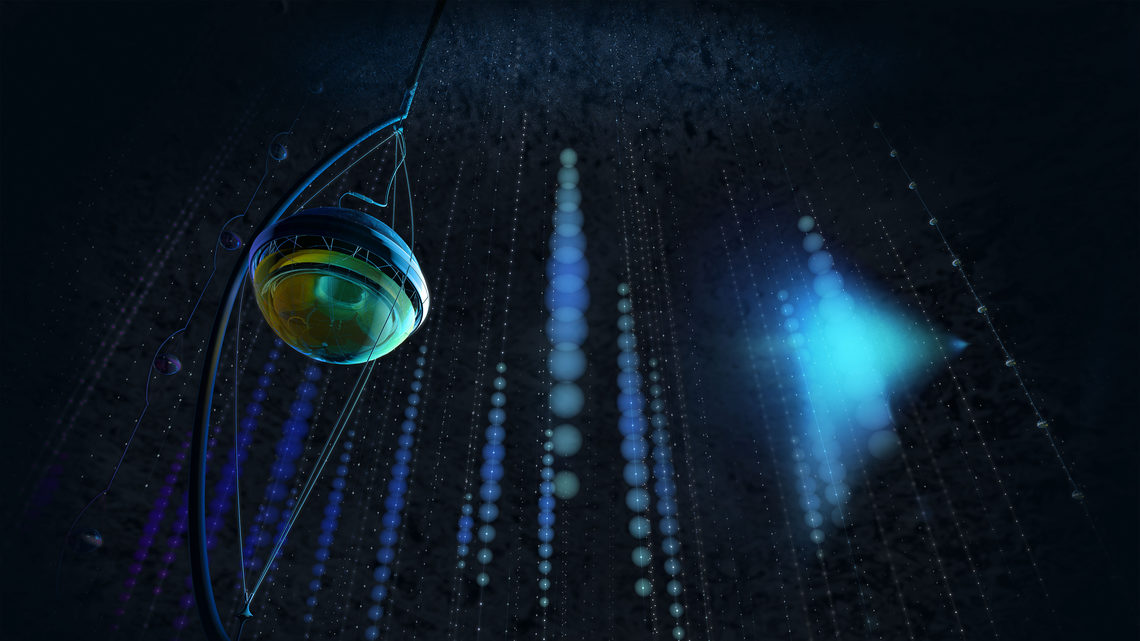
When cosmic neutrinos crash into the IceCube detector, the interactions generate secondary particles that travel faster than the speed of light through the ice, producing a detectable faint blue glow.
Extraterrestrial signals
One of the main aims of IceCube, which is run by an international group of more than 300 scientists from 12 different countries, is to identify cosmic neutrinos. They know which neutrinos come from afar by the extraordinarily high levels of energy they have when they crash into the Earth, compared to their more local counterparts. By studying these alien particles, physicists hope to identify the powerful cosmic accelerators that form beams of ultra-high energy particles.
IceCube had its first major breakthrough in 2013 when it identified two ultra-high energy neutrinos from outside the solar system. These events, dubbed Bert and Ernie, became the first of many cosmic neutrino detections, says Olga Botner, a physicist at Uppsala University in Sweden and former spokesperson of IceCube.
“We knew we were in business,” she says. “We could observe not only the atmosphere but also neutrinos from outside our own galaxy. That was huge.”
Four years later, IceCube physicists made a detection of an extraterrestrial neutrino that sparked a search for a glimpse of its source by scientists at astronomical observatories around the globe. This worldwide hunt allowed scientists to pinpoint the particle’s birthplace: an extremely luminous galaxy called a blazar. A blazar acts like a cosmic accelerator, spitting out a constant stream of particles from its core.
“Working on IceCube is very exciting,” says Delia Tosi, an assistant scientist at the Wisconsin IceCube Particle Astrophysics Center (WIPAC). “There is no space for boredom.”
Where most cosmic neutrinos come from remains a mystery. But IceCube’s scientific repertoire has expanded since those first discoveries. Scientists also use IceCube to examine how neutrinos change from one type to another—which could help determine whether there are new types of neutrinos that we don’t yet know about—as well as to search for dark matter and characterize how light travels though Antarctic ice.
“When we started IceCube, we were 90% focused on finding point sources of astrophysical neutrinos,” says Kael Hanson, a physicist and director of WIPAC. “We really had no idea, when we were designing the experiment, how rich the science program would eventually become.”
An upgrade on ice
With the forthcoming upgrade, more than 700 new sensors spread across seven strings will be added to the center of IceCube.
The core is already more densely packed with strings than the rest of the detector, which makes it better able to detect particles at low energies. The new sensors will push that sensitivity even further. “We’re pushing the energy threshold down by a factor of 10,” Hanson says.
The denser core will make it possible for the scientists to examine the hundreds of thousands of atmospheric neutrinos that bombard the detector each year in more detail. This will allow physicists to make more accurate measurements of the tau neutrino, which can then be used to better understand neutrino oscillations—specifically, how muon neutrinos convert to tau neutrinos.
“We don’t quite understand how neutrinos can spontaneously morph from one flavor to another,” Botner says. “If discrepancies exist between our predictions and what we observe, this would be a hint of unknown neutrino kinds—the so-called sterile neutrino.”
To insert new strings into the detector, scientists must drill deep holes into the ice using a high-pressure stream of hot water. During the upgrade, scientists will deploy additional calibration instruments, such as cameras and light sources, along with the detectors to help them characterize the ice.
When water refreezes around the strings—a process that can take several weeks—the ice that forms can contain dust and bubbles. These imperfections make it more difficult to see signs of neutrinos.
Not only will characterizing the ice make it possible for scientists to more accurately assess future observations, researchers will also be able to apply this new knowledge to previously collected data. “In principle, we can recalibrate all the data and improve our ability to point back to a source,” says Dawn Williams, a particle astrophysicist at the University of Alabama.
The IceCube collaboration plans to start drilling in late 2022. In the meantime, the group is preparing the sensors and other components of the upgrade as well as the software that will be used to run the upgraded detector. The team expects to start collecting data in the spring of 2023.
The upgrade also serves an additional purpose: to test new sensor designs that scientists hope might be deployed in IceCube-Gen2, a proposed detector that would be 10 times the size of the current one. The super-sized observatory would allow scientists to conduct even more precise measurements of neutrinos and detect more ultra-high-energy particles from outer space, heightening the possibly of pinpointing their sources.