Neutrinos are elementary particles first discovered six decades ago. Over the years, scientists have learned several surprising things about them. But they have yet to answer what might sound like a basic question: How much do neutrinos weigh? The answer could be key to understanding the nature of the strange particles and of our universe.
To understand why figuring out the mass of neutrinos is such a challenge, first you must understand that there’s more than one way to picture a neutrino.
Neutrinos come in three flavors: electron, muon and tau. When a neutrino hits a neutrino detector, a muon, electron or tau particle is produced. When you catch a neutrino accompanied by an electron, you call it an electron neutrino, and so on.
Knowing this, you might be forgiven for thinking that there are three types of neutrinos: electron neutrinos, muon neutrinos and tau neutrinos. But that’s not quite right.
That’s because every neutrino is actually a quantum superposition of all three flavors. Depending on the energy of a neutrino and where you catch it on its journey, it has a different likelihood of appearing as electron-flavored, muon-flavored or tau-flavored.
Armed with this additional insight, you might be forgiven for thinking that, when all is said and done, there is actually just one type of neutrino. But that’s even less right.
Scientists count three types of neutrino after all. Each one has a different mass and is a different mixture of the three neutrino flavors. These neutrino types are called the three neutrino mass states.
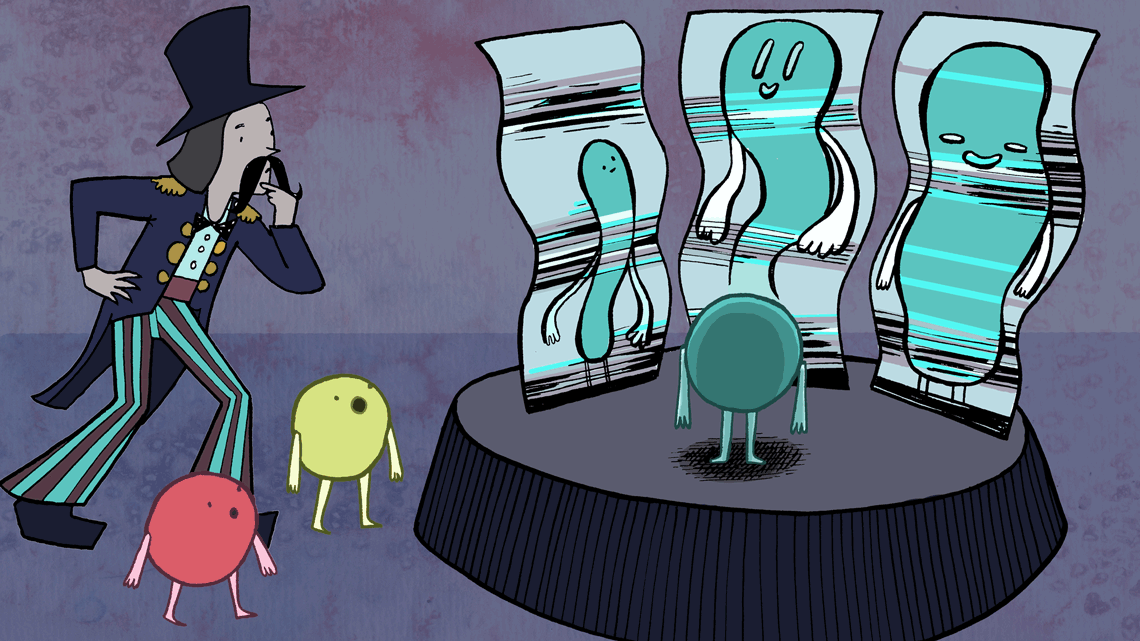
A weighty problem
We know that the masses of these three types of neutrinos are small. We know that the flavor mixture of the first neutrino mass state is heavy on electron flavor. We know that the second is more of an even blend of electron, muon and tau. And we know that the third is mostly muon and tau.
We know that the masses of the first two neutrinos are close together and that the third is the odd one out. What we don’t know is whether the third one is lighter or heavier than the others.
The question of whether this third mass state is the heaviest or the lightest mass state is called the neutrino mass hierarchy (or neutrino mass ordering) problem.
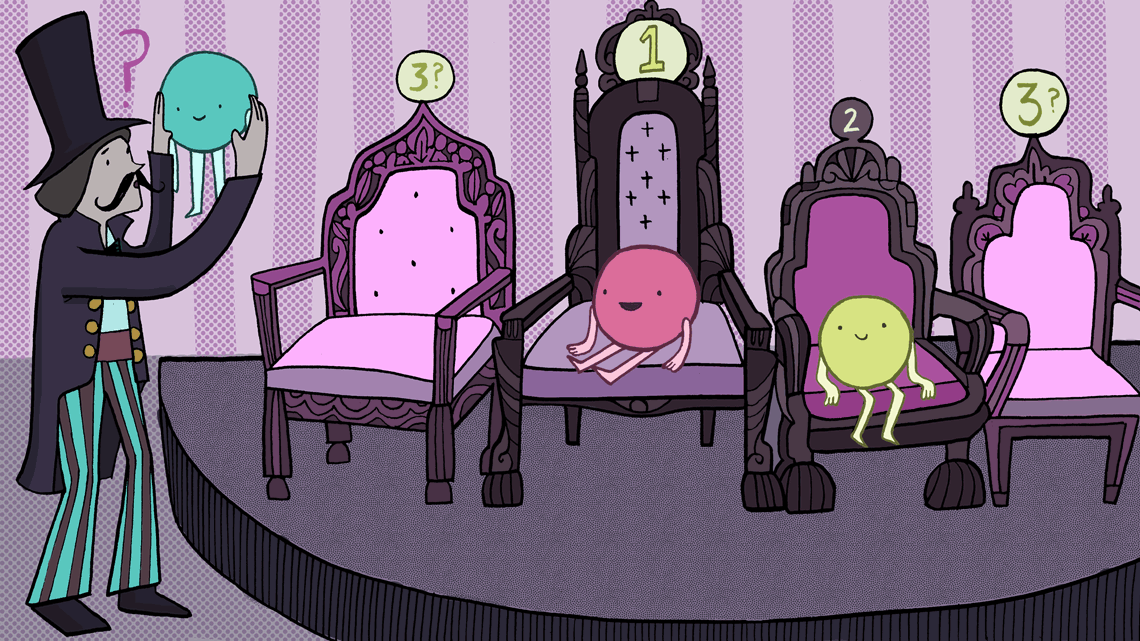
Easy as 1,2,3—or 3,1,2?
Some models that unify the different forces in the Standard Model of particle physics predict that the neutrino mass ordering will follow the pattern 1, 2, 3—what they call a normal hierarchy. Other models predict that the mass ordering will follow the pattern 3, 1, 2—an inverted hierarchy. Knowing whether the hierarchy is normal or inverted can help theorists answer other questions.
For example, four forces—the strong, weak, electromagnetic and gravitational forces—govern the interactions of the smallest building blocks of matter. Some theorists think that, in the early universe, these four forces were united into a single force. Most theories about the unification of forces predict a normal neutrino mass hierarchy.
Scientists’ current best tools for figuring out the neutrino mass hierarchy are long-baseline neutrino experiments, most notably one called NOvA.
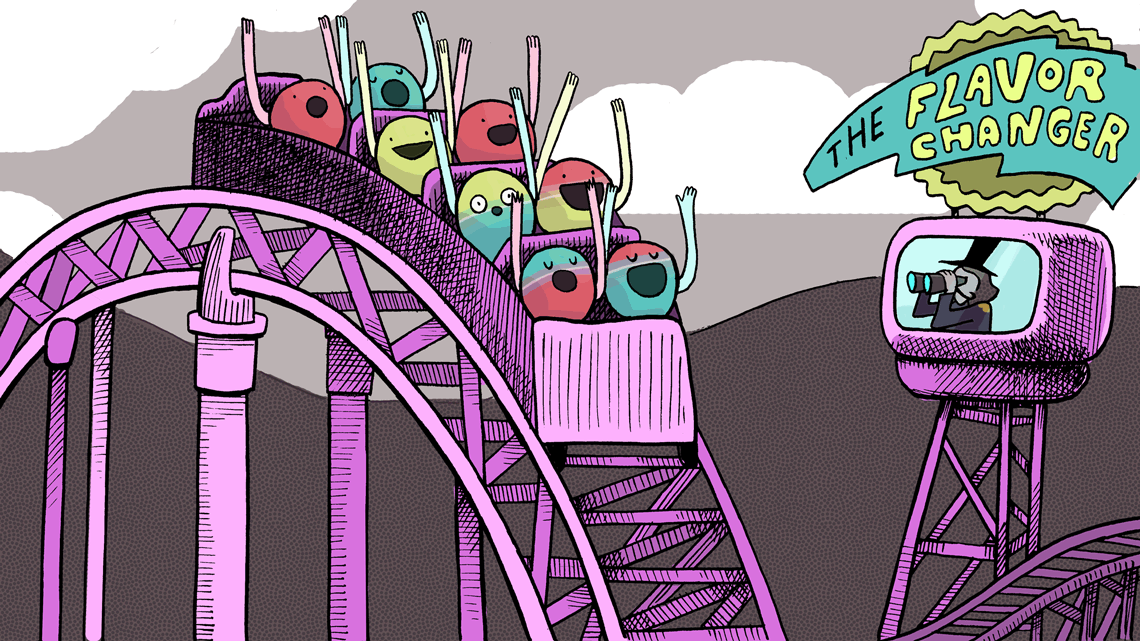
Electron drag
The NOvA detector, located in Minnesota near the border of Canada, studies a beam of neutrinos that originates at Fermi National Accelerator Laboratory in Illinois.
Neutrinos very rarely interact with other matter. That means they can travel 500 miles straight through the Earth from the source to the detector. In fact, it’s important that they do so, because as they travel, they pass through trillions of electrons.
This affects the electron-flavor neutrinos—and only the electron-flavor neutrinos—making them seem more massive. Since the first and second mass states contain more electron flavor than the third, those two experience the strongest electron interactions as they move through the Earth.
This interaction has different effects on neutrinos and antineutrinos—and the effects depend on the mass hierarchy. If the hierarchy is normal, muon neutrinos will be more likely to turn into electron neutrinos, and muon antineutrinos will be less likely to turn into electron antineutrinos. If the hierarchy is inverted, the opposite will happen.
So if NOvA scientists see that, after traveling through miles of rock and dirt, more muon neutrinos and fewer muon antineutrinos than expected have shifted flavors, it will be a sign the mass hierarchy is normal. If they see fewer muon neutrinos and more muon antineutrinos have shifted flavors, it will be a sign that the mass hierarchy is inverted.
The change is subtle. It will take years of data collection to get the first hint of an answer. Another, shorter long-baseline neutrino experiment, T2K, is taking related measurements. The JUNO experiment under construction in China aims to measure the mass hierarchy in a different way. The definitive measurement likely won’t come until the next generation of long-baseline experiments, DUNE in the US and the proposed Hyper-Kamiokande experiment in Japan.
Neutrinos are some of the most abundant particles in the universe. As we slowly uncover their secrets, they give us clues about how that universe works.